
Furthermore, such hydrogels’ hydrophilic nature, chemical stability and biodegradability lend favourably towards their use as versatile scaffolds for 3D printing of bio-synthetic tissue constructs using appropriate cells. Early work using materials such as chitosan have given way to more tissue-compliant hydrogels based on natural polymers, such as gelatine mainly due to their cytocompatibility and constitutional relevance to mammalian tissue 10. However, challenges still exist in the development of a fully functional tissue construct that can replicate its natural counterpart 8, 9.Īn important factor in chondral tissue engineering is the choice of biomaterial for scaffolds. In recent years this technology has inspired application for in vitro biofabrication of cartilage tissues 6, 7. The generation of organized 3D tissue constructs via a layer-by-layer deposition process that combines cells and biomaterials in an ordered and predetermined way, allows the fabrication of multi-cellular constructs where cell-cell and cell-material interactions can mimic the physiological environment and where cellular responses to stimuli are more reflective of those found in vivo 5. The ability to design and fabricate complex structures by printing living cells and biomaterials functionalized with biological molecules is revolutionizing tissue engineering and regenerative medicine 1, while enabling new possibilities in drug screening and toxicology 2, 3, 4. Three-dimensional (3D) bioprinting epitomizes the fusion of biology and engineering. Overall, the Core/Shell handheld 3D bioprinting strategy enabled rapid generation of high modulus bioscaffolds with high cell viability, with potential for in situ surgical cartilage engineering. This system allowed the generation of Core/Shell GelMa/HAMa bioscaffolds with stiffness of 200KPa, achieved after only 10 seconds of exposure to 700 mW/cm 2 of 365 nm UV-A, containing >90% viable stem cells that retained proliferative capacity.
#CORE SHELL SCAFFOLD FREE#
To fulfill those criteria, free radical cytotoxicity was confined by a co-axial Core/Shell separation. To progress towards translating this freeform biofabrication tool into clinical practice, we aimed to define the ideal bioprinting conditions that would deliver a scaffold with high cell viability and structural stiffness relevant for chondral repair. We recently developed a handheld 3D printer called “Biopen”.
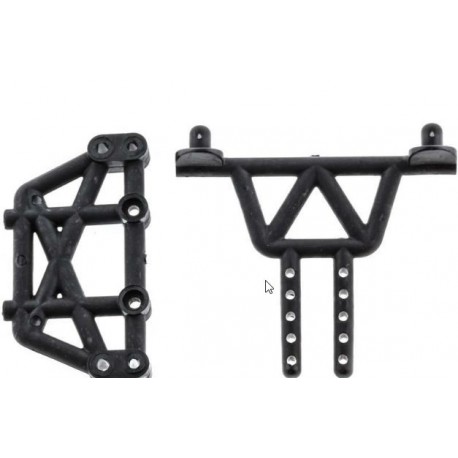
Therefore, the choice of the photocuring conditions has to be carefully addressed to generate a structure stiff enough to withstand the forces phisiologically applied on articular cartilage, while ensuring adequate cell survival for functional chondral repair. Extrusion-based 3D bioprinting necessitates a phase change from a liquid bioink to a semi-solid crosslinked network achieved by a photo-initiated free radical polymerization reaction that is known to be cytotoxic. Three-dimensional (3D) bioprinting is driving major innovations in the area of cartilage tissue engineering.
